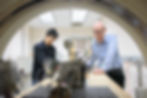
Research scientist Tae Wook Kim, left, and professor Tony Kovscek check the status of an experiment in the CT scanner. (Image credit: L.A. Cicero)
As the world continues to burn 100 million barrels of oil a day – a rate that is expected to continue for the next 50 years – Stanford Earth researchers are developing greener ways of extracting the oil and mitigating the resulting greenhouse gases.
Of the approximately two dozen medical CT scanners scattered throughout Stanford’s main campus and medical centers, two can be found nestled in basement labs of the Green Earth Sciences Buildings.
The scanner duo is being put to some decidedly off-label uses in research led by Anthony Kovscek, a professor of energy resources engineering at Stanford’s School of Earth, Energy & Environmental Sciences (Stanford Earth). The machines in this case have helped scientists extract oil and gas more efficiently, and are now revealing ways of storing carbon dioxide (CO2) deep underground while the world continues to rely on fossil fuels for energy and transportation.
The motorized tables that would normally slide patients in and out of the scanners’ openings instead support machines made from a daunting array of connected pressure vessels, nozzles and gauges shrouded beneath wires and tubing. The larger of the two scanners is about the length of a person and looks like something devised by a mad scientist to power a time-traveling DeLorean. “I’ll still sometimes say ‘Whoa’ when I see it,” said Muhammad Almajid, a graduate student in Kovscek’s lab.
The ad hoc devices, which Almajid and his colleagues painstakingly craft and assemble by hand, are designed to force pressurized oil, water or gas through slim cylinders of seemingly solid rock, which the scanners then analyze with X-rays.
“We try to visualize things that people say you can’t visualize,” said Kovscek, who is the Keleen and Carlton Beal Professor of Petroleum Engineering at Stanford. “It’s happened more than once, where someone will say, ‘Well, you just can’t do it.’ And I point to our results and say, ‘Well, I beg to differ.’”
The lab experiments are intended to recreate, in miniature, the movement of various substances through vast rock formations and to provide real-world validation of computer simulations of the same processes. This type of experimentation and simulation has helped the United States move toward energy security by enabling the nation to tap vast reserves of previously inaccessible oil and natural gas, such as shale oil.
But what’s learned from that mass of tubes and gauges could also help mitigate the effects of burning 100 million barrels of oil per day, which is expected to continue for at least 50 years while the world transitions to renewable energy sources. Results like the ones from Kovscek’s lab are now guiding new ways of sequestering the powerful greenhouse gas carbon dioxide, which is released from burning fossil fuels, deep within rocks, for eons, while avoiding leaks and other negative consequences – a strategy many experts say is going to be necessary in order to avoid the hazards of climate change.
“Greater efficiency in oil recovery and conversion of the energy system to renewables has to happen. But there is a multi-decade period where we really have to make sure that CO2 emissions are under control while hydrocarbons are still being consumed at high rates,” said Hamdi Tchelepi, a professor of energy resources engineering who frequently collaborates with Kovscek. “The only way to achieve that is through a serious, large-scale deployment of carbon sequestration. It’s not optional. In the eyes of the scientific community, this has to happen.”
Trapping carbon underground
Some of the strategies for efficiently extracting oil and natural gas from reservoirs that came out of work like Kovscek’s are also a first step toward carbon sequestration.
Especially relevant is a practice called enhanced oil recovery (EOR) by gas injection, which involves pumping pressurized gases into existing oil fields to displace or reduce the viscosity of crude oil, making it easier to extract. Even the best performing fields still leave about 50 percent of oil in the ground, Kovscek said. For unconventional resources such as shale rocks, which are even more difficult to extract oil from, the recovery rate can be as low as 5 percent.
“That means if we don’t do anything further on a well after the initial recovery, we’re going to leave as much as 95 percent of the resource in the ground,” Kovscek said. “That’s a huge waste of all the energy that it took to drill the well and the water injection and hydraulic fracturing that may have been required to operate it.”
CO2, it turns out, mixes very well with crude oil, making it, in petroleum engineering parlance, an excellent “EOR fluid.” Thus, for decades now, and for its own purposes, the fossil fuel industry has been refining the process of injecting huge amounts of CO2 deep underground.
The challenge for people like Kovscek and his colleagues is keeping that gas imprisoned underground and out of the atmosphere for long periods of time without unanticipated consequences.
“Oil companies are mostly interested in what’s called the active injection period because their goal is to get a return on investment within one or two decades,” Tchelepi said. “For sequestration, you need to inject CO2 for a couple of decades and then turn the valves off. And the physics of what happens after you stop injecting is more complicated than the physics used for oil recovery. You have to truly go a step beyond, because the time scales are so long.”
Understanding the dangers
A major focus of Tchelepi’s research is using 4-D computer simulations to predict how sequestered CO2 will interact with faults deep within the Earth across centuries and eons. “In some cases, we’ve modeled 3,000 to 4,000 years into the future,” Tchelepi said. “The ideal target for sequestration would be a large, reasonably homogenous rock basin that doesn’t have any fractures. But nature isn’t always so kind to us.”
The dangers associated with CO2 interacting wrongfully with faults are myriad. One risk is that the highly pressurized gas might nudge an already stressed fault toward its breaking point, generating earthquakes. Another risk that an existing crack is widened by the CO2, creating a pathway for other, more noxious gases to seep to the surface or pollute aquifers used for drinking and agriculture. “Carbon dioxide and water is Pellegrino [sparkling water],” Tchelepi said, “but it’s not pure Pellegrino, because CO2 usually contains harmful impurities that get generated when coal is burned but that aren’t fully removed before injection.”
Trying to minimize the risk of CO2 leakage is a focus of Lou Durlofsky’s research. Durlofsky, who is a professor of energy resources engineering, is adapting techniques from oil and gas applications to simulate the impacts of placing CO2 injection wells in different locations and varying the rates at which injection occurs. With powerful computer simulations, Durlofsky’s team can play virtual “what-if” games that allow it to determine well locations and injection sequences that nudge sequestered CO2 toward a desired fate.
The best-case scenario for trapped CO2 is for it to react with rock and form long-lasting minerals, but this process requires very long timescales to occur. However, there are two other favorable outcomes, which can happen much faster: dissolution, whereby the CO2 gets dissolved in salt water, and residual trapping, which is when CO2 gets broken up into very small bubbles that resist leakage.
“Because the CO2 is now in the form of discrete blobs, it can’t flow as easily and is effectively trapped in place,” explained Durlofsky, who is the Otto N. Miller Professor in Earth Sciences at Stanford. “By varying where and how CO2 gets injected in the formation, we can increase the likelihood that one or the other of these outcomes will occur.”
‘Green oil’
Despite the risks of leakage, Tchelepi said the results from Kovscek’s scanner experiments and his own team’s computer simulations indicate that safe, long-term carbon sequestration is within reach — and he thinks the technique should be deployed now, even though it’s still in its infancy. “It’s far from being perfected, but we know more than enough, in my opinion, to start using it,” Tchelepi said. “Clearly, there will be issues and problems, but the only way to deal with them is to put them under the control of science and engineering, to monitor them and to spend the resources to learn from the mistakes. The risk of waiting for perfection is too big. We know enough.”
One idea that Kovscek and Tchelepi’s labs are exploring is combining EOR and carbon sequestration to create what they refer to as “green oil.” “If you can take all of the CO2 that is generated from burning the oil or natural gas that’s extracted in the future from a reservoir, inject it back into the reservoir and store it securely, you would have net-zero carbon emissions,” Kovscek said. “Sequestration is expensive. If we can recover something valuable in the process, it can be used to pay for the sequestration.”
While some might see a contradiction in helping maximize the extraction of oil and natural gas, on the one hand, and working to sequester the CO2 created from the burning of those same fuels on the other, Tchelepi views the two goals as complementary.
“You have to be realistic that we will use 100 million barrels of oil a day for the next 50 years,” he said. “Should we do that in a messy, uncontrolled way? Or should we do it with the best possible engineering, maximize the recovery and optimize it by coupling it with sequestration? I’m working on the second option.”
Stanford Earth energy resources engineering professors Anthony Kovscek, Hamdi Tchelepi and Lou Durlofsky are affiliate members of Stanford’s Precourt Institute for Energy.